Introduction
In cell-based regenerative therapies, transplantation
of cells into target tissues usually takes place
after cell manipulation, but most such manipulations
(e.g., cell culture) require animal-derived products
like serum or tissue extracts. Considering the risks,
which include infection with viral or prion-related
disease or immunological reactions,1 use of animal-derived
products like fetal bovine serum (FBS) or bovine
pituitary extract should be avoided. Thus, human-derived
substances are considered the optimum materials
for these manipulations. Autogolous serum obtained
from whole blood enhances the expansion of human
mesenchymal stem cells in culture.2-6 On the other
hand, preparation for clinical use of component-level
plasma products, such as platelet-rich plasma (PRP)
or platelet-poor plasma (PPP), is considered to
be less invasive because erythrocytes can be separately
collected in the form of “high-density erythrocytes,”
which can be given back to the patients.7,8
The proliferative effects in culture of various
platelet derivatives like PRP, platelet-released
supernatant, and platelet lysates on several human
cell types have been reported.6,9-14 Our aim was
to investigate the differences among three types
of autologous serum?serum from whole blood (SWB),
serum from PRP (SPRP), and serum from PPP (SPPP)?in
their effects on the growth of three representative
replicating human cells: human adipose-derived stem/stromal
cells (hASCs), human dermal fibroblasts (hDFs),
and human umbilical vein endothelial cells (HUVECs).
It is well known that the bioactive protein levels
secreted by platelets substantially differ among
donors, platelet product types, processing techniques,
and methods of platelet activation.15-21 Therefore,
in this study, SWB, SPPP, and SPRP were prepared
from the same four volunteers and evaluated for
biochemical components and clinical potential as
culture additives.
Materials and Methods
Collection and preparation of plasma and serum
Venous blood (300 mL each) was collected from four
healthy volunteers after informed consent approved
by our institutional review board (IRB). For preparation
of PRP and PPP, the methods clinically applied for
the preparations of autologous blood transfusion
in our facility were used. The preparation protocol
and blood components of whole blood (WB), PRP, and
PPP are summarized in Figure 1A and B. Serum was
prepared from WB, PRP, or PPP by elimination of
coagulation factors such as fibrinogen, as described
below.
First, 100 mL of each blood sample was drawn into
a flask, and the remaining 200 mL of blood was drawn
and stored in a blood bag (blood bag CPDA, Terumo,
Tokyo, Japan) containing 0.327% citric acid, 2.63%
sodium citrate, 0.0275% adenine, 0.251% sodium dihydrogen
phosphate, and 2.9% D-glucose solution.
The blood in the flask was oscillated (agitated)
at 37°C for one hour and incubated overnight at
4°C. The supernatant was collected using a 50-mL
tube and centrifuged at 841 ?g for 10 minutes using
a desktop centrifuge (KUBOTA 5200, Kubota, Co.,
Tokyo, Japan), and the supernatant was again collected
as SWB. The stored 200 mL of blood in the blood
bag was separated into 100 mL aliquots; one was
centrifuged at 93 ?g for 10 minutes and the other
at 841 ?g for 10 minutes. The resulting supernatants
were PRP and PPP, respectively. The two types of
plasma, PRP and PPP, were drawn into two flasks.
After addition of 200 U of thrombin, the contents
were oscillated (agitated) for 60 minutes at 37°C
and incubated overnight at 4°C. The liquid component
was drawn into a 50-mL tube and centrifuged at 841
?g for 10 min, and the supernatants were obtained
as SPRP and SPPP, respectively. The serum samples
were frozen at -80°C and thawed at 37°C before analysis.
Biochemical analysis
A small portion of WB, PRP, and PPP was used for
biochemical analysis to investigate the number of
red blood cells (RBCs), white blood cells (WBCs),
platelets, total protein (TP), albumin (Alb), sodium
(Na+), potassium (K+), chloride (Cl-), and calcium
(Ca++). Analysis was performed by SRL, Inc. (Tachikawa,
Japan), a commercial analysis service.
Quantitative analysis of platelet-originated
growth factors contained in serum
To analyze the concentration of platelet-originated
growth factors in each serum sample, platelet-derived
growth factor (PDGF) and epidermal growth factor
(EGF) were measured using respective anti-human
ELISA kits (Quantikine, R&D Systems, Inc., MN)
according to the manufacturer’s instructions. Levels
of immunoreactive cytokines were measured at 450
nm by a microplate reader (Bio-Rad Laboratories
Model 550, Hercules, CA), and a standard curve was
generated to determine growth factor concentrations
(pg/mL).
Preparation of human dermal fibroblasts
(hDFs)
Human dermal fibroblasts (hDFs) were isolated from
normal skin samples obtained from plastic surgery
after informed consent approved by the IRB. The
skin samples were cut into pieces of approximately
3 ? 3 mm in size and treated with 0.25% trypsin
in phosphate buffered saline (PBS) solution for
24 hours at 4°C. After removal of the epidermis,
the interstitial tissue fragments were attached
to 100-mm plastic dishes, and cultured with DMEM
(Nissui Pharmacertical, Tokyo, Japan) culture medium
containing 10% FBS. Primary hDFs appeared in 4 to
7 days around the interstitial tissue fragments
(after the initiation of outgrowth cultures) and
became confluent after 2 to 3 weeks.
Preparation of human adipose-derived
stem/stromal cells (hASCs)
Informed consent was obtained from each participant
before collection of lipoaspirates from body-contouring
surgery, according to the IRB-approved protocol.
Human ASCs were isolated from the samples and cultured
as previously described.22 In brief, the suctioned
fat was digested with 0.075% collagenase in PBS
solution for 30 minutes with agitation at 37°C.
Mature adipocytes and connective tissues were separated
from pellets by centrifugation at 800 ?g for 10
minutes. Cell pellets were passed through a 100-μm
mesh filter (Millipore, MA) to remove debris and
plated at a density of 5 ? 106 nucleated cells/100-mm
plastic dish. Cells were cultured in M-199 medium
containing 10% FBS at 37°C under 5% CO2 in a humidified
incubator.
Preparation of human umbilical
vein endothelial cells (HUVECs)
Before obtaining the placenta and umbilical cord
samples, informed consent was obtained from each
participant according to the protocol approved by
IRB. Isolation and culture of HUVECs was done according
to the method described by Jaffe et al.23 Samples
were immediately collected after delivery, separating
the umbilical cord from the placenta by clipping
both ends, and irrigated using 1% iodine/PBS solution.
To eliminate iodine, the intracelial space was rinsed
using M-199 medium and filled with 0.25% trypsin
in PBS. Both ends were again clipped, followed by
incubation for 10 minutes at 37°C. Then, the intracelial
space was rinsed using endothelial basal medium
(EBM; Cambrex, Walkersville, MD), and cells were
collected. The cells were centrifuged at 450 ?g
for 5 minutes, attached to 100-mm plastic dishes,
and cultured with EBM medium containing 2% FBS.
Cell proliferation assay using
culture medium containing various sera
Standard culture media were prepared (DMEM for hDFs,
M199 for hASCs, and EBM for HUVEC) containing FBS,
SWB, SPRP, or SPPP at concentrations of 0%, 5%,
and 10% for hDFs and hASCs, and of 0%, 1%, and 2%
for HUVEC, respectively. A total of 5 ? 104 cells
were plated in 60-mm dishes containing the prepared
medium, and the medium was changed on the third
and fifth days. Cells were counted on day 7 using
a cell counter (NucleoCounter, Chemometec, Co.,
Allerod, Denmark). The average numbers were calculated
from three different cultures for each cell type
and culture condition.
Statistical analysis
Results were expressed as mean ± SE (standard error).
To compare blood cell count and biochemical data
for each sera, the values of SPRP and SPPP were
described as a ratio to those of SWB. The Student’s
unpaired t-test was used to evaluate the differences
in influences on cell proliferation between FBS
and human sera, while the Student’s paired t-test
was used for different types of human sera. No correction
was made for multiple comparisons. Statistical significance
was defined as p < 0.05.
Results
Blood cell count and biochemical analysis of WB,
PRP, and PPP
The numbers of RBCs, WBCs, and platelets, and levels
of total protein and albumin in WB at the time of
blood collection were 428.0 (± 23.7) × 104 /μl,
6,100.0 ± 980.6 /μl, 25.0 (± 1.5) × 104 /μl, 7.0
± 0.4 g/dl, and 4.2 ± 0.4 g/dl, respectively. As
shown in Figure 2, in PRP, 75.1% of platelets remained
in comparison with WB, although RBCs and WBCs decreased
to 0.6% and 7.1%, respectively. In PPP, the number
of platelets decreased to 12.6% with a decrement
of RBCs and WBCs to 0.35% and 2.2%, respectively.
Despite these reductions in blood cell numbers,
the values for total protein and albumin were relatively
uniform. In addition, we identified no notable changes
in electrolyte levels.
PDGF and EGF concentrations in
SWB, SPRP, and SPPP
Concentrations of PDGF and EGF were quantitatively
analyzed by ELISA. As shown in Figure 3, in comparison
with the values for SWB, SPRP contained 86.5% and
93.5% of PDGF and EGF respectively, while SPPP included
only 19.1% and 11.2 % of those growth factors, respectively.
Effects of SWB, SPRP, and SPPP
on the proliferation of various cell types
The number of proliferated cells in the culture
media with different serum types at various concentrations
was compared to that obtained by culture with FBS
at the same concentration, using hDFs, hASCs, and
HUVECs. Although the degree differed among the cell
types, human-originated sera were effective for
the expansion of cell number by culture.
In the hDF culture, SWB and SPRP exhibited a high
proliferative efficacy that was almost identical
to that of FBS, although cells cultured in SPPP
showed a significantly lower degree of proliferation
compared to other sera (Fig. 4A). Representative
microscopic views of cultured hDFs are shown in
Figure 4B.
In the hASC culture, although with the addition
of SWB or SPRP cell proliferation outcome was inferior
to that for FBS, the efficacy of cell proliferation
was enhanced with increasing concentration of serum
human products. There was no significant difference
among effects of SWB, SPRP, and SPPP. Representative
microscopic views of cultured hASCs are shown in
Figure 5B.
Proliferation of HUVECs with SWB, SPRP, or SPPP
did not significantly differ among the three types
of human sera and was not as robust as that which
occurred with addition of FBS (Fig. 6A). Representative
microscopic views of cultured HUVECs are shown in
Figure 6B.
Discussion
Because specific gravity differs among various blood
components, we can isolate each one by a specific
centrifugation protocol; however, cell contamination
cannot be completely avoided because of slight overlaps
among these specific gravities. With our separation
protocol, subtraction of RBCs and WBCs was sufficient
in both PRP and PPP, and platelets were successfully
preserved in PRP compared to PPP. Specifically regarding
RBCs, less than 1% of the original numbers in WB
remained in PRP or PPP. An expected advantage in
the future use of these component-level serum (or
plasma) products in regenerative medicine lies primarily
in the possibility of salvage use of RBCs, conferring
greater interest in SPRP and SPPP than in SWB.
Secretory proteins such as PDGF, EGF, transforming
growth factor (TGF)-β1, and vascular endothelial
growth factor (VEGF) are stored in the α-granules
of platelets and released by platelet activation
via addition of thrombin17,20 or adenosine diphosphate,20
or by a freeze/thaw cycle.11,16 Preparation and
activation methods influence secretory protein concentrations.18,21
Platelet activation with thrombin, which we used
in this study, is considered to closely imitate
the physiologic activation of platelets, ensuring
the bioactivity of secreted growth factors.6,24
In the present study, the concentration of total
protein and albumin decreased slightly in the separation
process of PRP and PPP from WB, but the concentrations
of PDGF and EGF significantly decreased in proportion
to the reduction in platelet count. Platelet-derived
growth factors and platelet count were considered
to be intimately associated, although the alteration
was not linear.15,16,19
SWB and SPRP showed a high proliferative effect
on hDFs, an effect almost identical to that of FBS,
while hDFs cultured in SPPP showed a significantly
lower degree of proliferation. Some platelet-originated
growth factors, such as PDGF, are notable mitogens
for hDFs.19,25 The difference in hDF proliferation
effects among SWB, SPRP, and SPPP may primarily
arise from differences in concentrations of the
platelet-originated growth factors.
In ASC culture, although cell proliferation was
generally enhanced depending on the concentrations
of the three human serum preparations, cell proliferation
outcome was inferior to that achieved with FBS.
Our results using human sera obtained from the same
four donors are inconsistent with the previous finding
of Kocaoemer et al.6 that the proliferative efficacies
of pooled human AB serum (corresponding to SWB in
our study) and thrombin-activated PRP (corresponding
to SPRP in our study) surpassed that of FBS. Platelets
do not provide some major growth factors, such as
basic fibroblast growth factor (b-FGF), keratinocyte
growth factor, and hepatocyte growth factor.7,26
This may be the reason that human platelet-originated
growth factors are not sufficient for expansion
of hASCs; FBS may contain ingredients more influential
for hASC proliferation, such as b-FGF.
For manipulating stem cells in regenerative medicine,
differentiation capacity should be considered as
well as proliferation capacity, and an optimal culture
additive differs according to the purpose of the
culture. Because PDGF is known to be a potent inhibitor
of adipogenic differentiation of hASCs, SPPP with
a selective addition of recombinant growth factors
such as b-FGF and/or EGF may be preferable to SWB
in hASC culture for adipose tissue engineering.14,27
In our study, differentiation capacity after cell
expansion was not assessed because of the volume
limitation of the samples.
In HUVEC culture, cell growth with either FBS or
human serum preparations was inferior to that in
a specific endothelial growth medium (data not shown),
probably because growth factors such as b-FGF and
VEGF that are not sufficiently present in serum
are critical factors for HUVEC proliferation. The
results of all human serum preparations were significantly
worse than those obtained with FBS, although the
three human serum preparations showed no significant
differences among one another. A supplemental use
of angiogenic growth factors may enhance the proliferative
effect of serum products on HUVECs.
To our knowledge, this study is the first to compare
different human serum preparations as an additive
of cell culture, using blood samples obtained from
identical donors. We found that SWB and SPRP are
superior to SPPP as substitutes for animal-derived
serum in culture expansion of hDFs. Platelet-derived
ingredients, however, are considered non-essential
or insufficient for enhanced proliferation of hASCs
and HUVECs. Although autologous or human-derived
serum preparations may be of great use in cell-based
therapies in the future, this usefulness strongly
depends on the target cell species and the purpose
of the cell culture. Future studies should focus
on establishing the optimal indications of each
human serum preparation.
References
1. Tuschong, L., Soenen, S. L.,
Blaese, R. M., et al. Immune response to fetal calf
serum by two adenosine deaminase-deficient patients
after T cell gene therapy. Hum. Gene Ther. 13: 1605,
2002.
2. McAlinden, M. G., Wilson, D.
J. Comparison of cancellous bone-derived cell proliferation
in autologous human and fetal bovine serum. Cell
Transplant. 9: 445, 2000.
3. Stute, N., Holtz, K., Bubenheim,
M. et al. Autologous serum for isolation and expansion
of human mesenchymal stem cells for clinical use.
Exp. Hematol. 32: 1212, 2004.
4. Shahdadfar, A., Fronsdal, K.,
Haug, T. et al. In vitro expansion of human mesenchymal
stem cells: choice of serum is a determinant of
cell proliferation, differentiation, gene expression,
and transcriptome stability. Stem Cells 23: 1357,
2005.
5. Anselme, K., Broux, O., Noel,
B., et al. In vitro control of human bone marrow
stromal cells for bone tissue engineering. Tissue
Eng. 8: 941, 2002.
6. Kocaoemer, A., Kern, S., Kluter,
H., et al. Human AB serum and thrombin-activated
platelet-rich plasma are suitable alternatives to
fetal calf serum for the expansion of mesenchymal
stem cells from adipose tissue. Stem Cells 25: 1270,
2007.
7. Sanchez, A. R., Sheridan, P.
J., and Kupp, L.I. Is platelet-rich plasma the perfect
enhancement factor? A current review. Int. J. Oral
Maxillofac. Implants. 18: 93, 2003.
8. Galel, S. A., Malone, III J.
M., Viele, M. K. Transfusion medicine. In Wintrobe’s
clinical hematology, 11th edition. Philadelphia:
Lippincott Williams & Wilkins, 2004.
9. Lucarelli, E., Beccheroni, A.,
Donati, D., et al. Platelet-derived growth factors
enhance proliferation of human stromal stem cells.
Biomaterials 24: 3095, 2003.
10. Gruber, R., Karreth, F., Kandler,
B., et al. Platelet-released supernatants increase
migration and proliferation, and decrease osteogenic
differentiation of bone marrow-derived mesenchymal
progenitor cells under in vitro conditions. Platelets
15: 29, 2004.
11. Doucet, C., Ernou, I., Zhang,
Y., et al. Platelet lysates promote mesenchymal
stem cell expansion: a safety substitute for animal
serum in cell-based therapy applications. J. Cell.
Physiol. 205: 228, 2005.
12. Choi, B. H., Zhu, S. J., Kim,
B. Y., et al. Effect of platelet-rich plasma (PRP)
concentration on the viability and proliferation
of alveolar bone cells: an in vitro study. Int.
J. Oral Maxillofac. Surg. 34: 420, 2005.
13. Vogel, J. P., Szalay, K., Geiger,
F., et al. Platelet-rich plasma improves expansion
of human mesenchymal stem cells and retains differentiation
capacity and in vivo bone formation in calcium phosphate
ceramics. Platelets 17: 462, 2006.
14. Koellensperger, E., von Heimburg,
D., Markowicz, M., et al. Human serum from platelet-poor
plasma for the culture of primary human preadipocytes.
Stem Cells 24: 1218, 2006.
15. Marx, R. E. Platelet-rich plasma
(PRP): what is PRP and what is not PRP? Implant.
Dent. 10: 225, 2001.
16. Weibrich, G., Kleis, W. K.,
Hafner, G., et al. Growth factor levels in platelet-rich
plasma and correlations with donor age, sex, and
platelet count. J. Craniomaxillofac. Surg. 30: 97,
2002.
17. Gonshor, A. Technique for producing
platelet-rich plasma and platelet concentrate: background
and process. Int. J. Periodontics. Restorative.
Dent. 22: 547, 2002.
18. Zimmermann, R., Arnold, D.,
Strasser, E., et al. Sample preparation technique
and white cell content influence the detectable
levels of growth factors in platelet concentrates.
Vox Sang 85: 283, 2003.
19. Eppley, B. L., Woodell, J.
E., and Higgins, J. Platelet quantification and
growth factor analysis from platelet-rich plasma:
implications for wound healing. Plast. Reconstr.
Surg. 114: 1502, 2004.
20. Eppley, B. L., Pietrzak, W.S.,
and Blanton, M. Platelet-rich plasma: a review of
biology and applications in plastic surgery. Plast.
Reconstr. Surg. 118: 147e, 2006
21. Everts, P. A., Brown, Mahoney,
C., Hoffmann, J. J., et al. Platelet-rich plasma
preparation using three devices: implications for
platelet activation and platelet growth factor release.
Growth Factors 24: 165, 2006.
22. Yoshimura, K., Shigeura, T.,
Matsumoto, D., et al. Characterization of freshly
isolated and cultured cells derived from the fatty
and fluid portions of liposuction aspirates. J.
Cell. Physiol. 208: 64, 2006.
23. Jaffe, E. A., Nachman, R. L.,
Becker, C. G., et al. Culture of human endothelial
cells derived from umbilical veins. Identification
by morphologic and immunologic criteria. J. Clin.
Invest. 52: 2745, 1973.
24. Marx, R. E. Platelet-rich plasma:
Evidence to suport its use. J. Oral Maxillofac.
Surg. 62: 489, 2004.
25. Martin, P. Wound healing-Aiming
for perfect skin regeneration. Science 276: 75,
1997.
26. Aiba-Kojima, E., Tsuno, N.
H., Inoue, K., et al. Characterization of wound
drainage fluids as a source of soluble factors associated
with wound healing: comparison with platelet-rich
plasma and potential use in cell culture. Wound.
Repair. Regen. 15: 511, 2007.
27. Hauner, H., Rohrig, K., and
Petruschke, T. Effects of epidermal growth factor
(EGF), platelet-derived growth factor (PDGF) and
fibroblast growth factor (FGF) on human adipocyte
development and function. Eur. J. Clin. Invest.
25: 90, 1995.
Figure legends
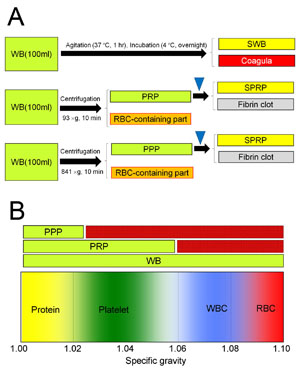
Fig. 1. Preparation of three types of human serum
(A) For preparation of PRP or PPP, WB is separated
by centrifugation into two components; PRP and the
remainder (the RBC-containing part), or PPP and
the remainder (the RBC-containing part). SPRP and
SPPP were prepared through thrombin activation (blue
triangle) of PRP and PPP, respectively.
(B) Blood components differ in specific gravity.
Because of the differential specific gravity, PRP
and PPP can be separated from WB by specific centrifugation
protocols.
WB: whole blood, PRP: platelet-rich plasma, PPP:
platelet-poor plasma, SWB: serum from WB, SPRP:
serum from PRP, SPPP: serum from PPP, WBC: white
blood cells, RBC: red blood cells.
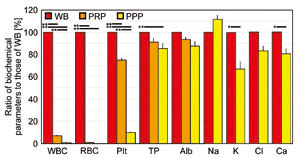
Fig. 2. Blood counts and biochemical data in WB,
PRP, and PPP
Values are expressed as ratios of biochemical parameters
to those of WB. Student’s paired t-tests were used
for statistical analysis. Black bars above indicate
statistical significance (*p < 0.05, **p <
0.01). Values are mean, SE.
WB: whole blood, PRP: platelet-rich plasma, PPP:
platelet-poor plasma, WBC: white blood cells, RBC:
red blood cells, TP: total protein, Alb: albumin.
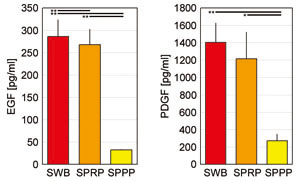
Fig. 3. Concentrations of platelet-derived cytokines
in SWB, SPRP, and SPPP
Concentrations of two representative platelet-derived
growth factors, EGF and PDGF, were measured in three
types of serum prepared using various centrifugation
conditions. Error bars indicate SE. Student’s paired
t-tests were used for statistical analysis (*p <
0.05, ** p < 0.01).
SWB: serum from whole blood, SPRP: serum from platelet-rich
plasma, SPPP: serum from platelet-poor plasma, EGF:
epidermal growth factor, PDGF: platelet-derived
growth factor.
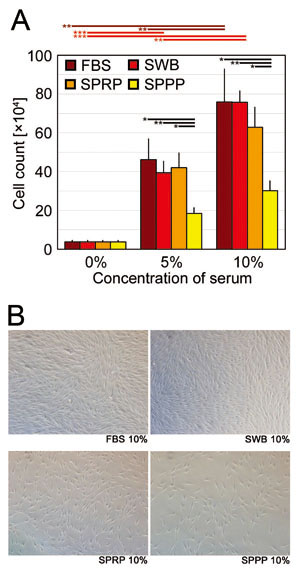
Fig. 4. Human-dermal fibroblast (hDF) proliferation
assay
(A) Cell counts of hDFs on day 7 of culture with
addition of FBS, SWB, SPRP, or SPPP at concentrations
of 0%, 5%, and 10%. Error bars indicate SE. Student’s
paired t-tests were used for statistical analysis.
Horizontal bars indicate statistical significance
between different concentrations of each serum,
or between different types of serum at the same
concentration (*p < 0.05, **p < 0.01, ***p
< 0.001).
(B) Representative microscopic views of hDFs (day
7) cultured with 10% FBS, SWB, SPRP, or SPPP.
FBS: fetal bovine serum, SWB: serum from whole blood,
SPRP: serum from platelet-rich plasma, SPPP: serum
from platelet-poor plasma.
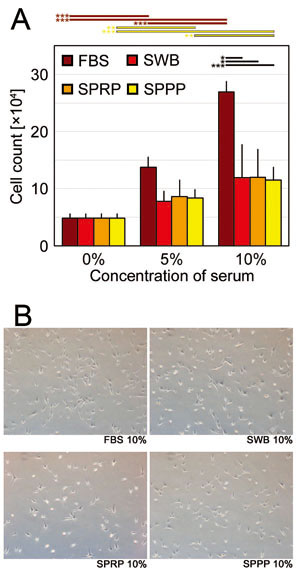
Fig. 5 Human adipose-derived stem/stromal cell (hASC)
proliferation assay
(A) Cell numbers of hASCs on day 7 cultured with
addition of FBS, SWB, SPRP, or SPPP at concentrations
of 0%, 5%, and 10%. Error bars indicate SE. Student’s
t-tests were used for statistical analysis. Horizontal
bars indicate statistical significance between different
concentrations of each serum, or between different
types of serum at the same concentration (*p <
0.05, **p < 0.01, ***p < 0.001).
(B) Representative microscopic views of hASCs (day
7) cultured with 10% FBS, SWB, SPRP, or SPPP.
FBS: fetal bovine serum, SWB: serum from whole blood,
SPRP: serum from platelet-rich plasma, SPPP: serum
from platelet-poor plasma.
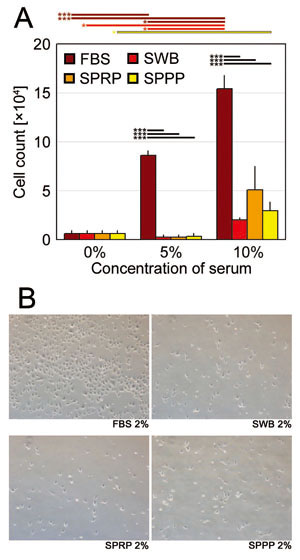
Fig. 6. Human umbilical vein endothelial cell (HUVEC)
proliferation assay
(A) Numbers of HUVECs on day 7 cultured with FBS,
SWB, SPRP, or SPPP at concentrations of 0%, 1%,
and 2%. Error bars indicate SE. Student’s paired
t-tests were used for statistical analysis. Horizontal
bars indicate statistical significance between different
concentrations of each serum, or between different
types of serum at the same concentration (*p <
0.05, **p < 0.01, ***p < 0.001).
(B) Representative microscopic views of HUVECs (day
7) cultured with 10% FBS, SWB, SPRP, or SPPP.
FBS: fetal bovine serum, SWB: serum from whole blood,
SPRP: serum from platelet-rich plasma, SPPP: serum
from platelet-poor plasma.