Introduction
Human adipose-derived stem (stromal) cells (ASCs)1,2
are promising as a tool of regenerative therapies
for tissue defects of mesenchymal lineage, including
fat,3 bone,4-8 and cartilage,9-11 as well as blood
vessels.12-14 As emphasized for other types of stem/progenitor
cells,15 harvested autologous ASCs will most probably
be preserved for multiple clinical applications
in the future. Cryopreservation confers many advantages
for practitioners engaged in cell-based therapies,
including transportability of stem cells, pooling
of cells to reach a therapeutic dose, and time for
the completion of safety and quality control testing.15
Therefore, cryopreservation of ASCs should be intensively
investigated for optimization as an indispensable
fundamental technology. As the first step to achieving
this aim, we examined the proliferative capacity
and multipotency of human ASCs before and after
long-term (6 months) cryopreservation under our
defined protocol.
Materials and Methods
Human tissue samples
ASCs were harvested at surgery from lipoaspirates
of 14 female patients, ages 20 to 51 years (mean,
29.6 years). Donor sites were abdomen and/or thighs.
Doubling times were determined for ASCs from five
patients before cryopreservation and from another
four patients after 6 months of cryopreservation;
therefore, the cell lines for the assays before
and after cryopreservation were not identical. Similarly,
four lines of ASCs were assayed for determination
of chondrogenic potential with the micromass culture
system (see below) before cryopreservation, while
another five lines were examined after cryopreservation.
For osteogenic and adipogenic differentiation assays,
five lines of ASCs were assayed before cryopreservation,
while a distinct four lines were examined after
cryopreservation. Of these five and four lines,
one in the before and after groups was from the
same patient, and the others were all from separate
patients. As for flow cytometry, we analyzed the
expression of a set of surface markers (see below)
of four lines of ASCs before cryopreservation and
five lines after cryopreservation; one patient in
both before and after; four other patients in the
before, and three other patients in the after.
Cell isolation and culture
All protocols used for these procedures have been
described previously.16
Cryopreservation of cells
ASCs were counted and resuspended in cryoprotective
agent (Cell Banker IR, Wako Chemicals Co., Ltd.,
Osaka, Japan) at a density of 106 cells/mL. They
were then frozen under temperature control using
a programmed freezer (PROFREEZE TNP-87S2Q, Nihon
Freezer Co., Ltd., Tokyo, Japan) under a protocol
of 4°C for 5 min, then decreasing ?1°C/min until
temperature reached ?50°C, followed by a decrease
of ?5°C/min until ?80°C. Samples were then transferred
to a liquid nitrogen tank (Isothermal Vapor Storage
System V-1500 with Series 2300 Auto-fill/Monitor
System, Custom Biogenic Systems, MI) for long-term
storage at ?196°C.. Cells before cryopreservation
were subjected to each assay at passage 1 or 2,
while those after 6 months of cryopreservation underwent
the assays at passage 2 or 3. Cells that had been
cryopreserved for 6 months were rapidly thawed in
a warm water bath set at 37°C, spun to remove the
cryoprotective agent, and seeded onto appropriate
culture plates for each experiment.
Measurement of doubling time
Fresh or cryopreserved ASCs were seeded onto six-well
plates at a density of 1 × 105 cells/well and allowed
to grow until they reached logarithmic growth phase.
It usually took 3 days for cells to start proliferating
logarithmically, but sometimes (especially at passage
0) it took 5?6 days. The cells were then sequentially
trypsinized at intervals of 48 hours and counted
with a cell counter (NucleoCounter, Chemometec,
Denmark). Doubling time was calculated according
to the following formula: doubling time = 48 hours/log2(N2/N1),
where N1 is the first cell count and N2 is the cell
count 48 hours later.
Induced differentiation of cultured
ASCs
The protocol for chondrogenic differentiation (micromass
culture system) has been described previously.17
DMEM/10% FBS was used for the culture medium as
negative control. Quantification was done by measuring
the micromass sizes on microphotographs with a scale
using an image analysis software (Scion v.3.53,
Scion Corporation, MA). Safranin O staining was
performed for qualitative analysis.
To test osteogenic differentiation, calcium deposition
was evaluated based on the ortho-cresolphthalein
complexone (OCPC) method18 with the Calcium C-Test
Wako Kit (Wako Chemicals) according to the manufacturer’s
instructions. After 5 × 104 cells/60-mm dish were
cultured in M199 containing 10% FBS for 6 days,
the medium was replaced with osteogenic differentiation
medium (DMEM containing 10% FBS, 50 μM ascorbate-2-phosphate,
0.1 μM dexamethasone, and 10 mM β-glycerophosphate)
or control medium (DMEM with 10% FBS). Cells were
then assayed after 1, 2, 3, and 4 weeks of differentiation
culture.
For assessment of adipogenic differentiation, cells
were seeded onto 96-well plates at a density of
1 × 104 cells/well. After 4 days of culture, medium
was replaced with adipogenic differentiation medium
(DMEM with 10% FBS, 0.5 mM isobutylmethylxanthine,
1 μM dexamethasone, 10 μM insulin, and 200 μM indomethacin)
or control medium (DMEM with 10% FBS). Cells were
further cultured for 1, 2, 3, or 4 weeks and stained
with 5 μL/well of AdipoRed-Lipid Assay ReagentR
(Cambrex, NJ). After 10 minutes of incubation, the
fluorescence with excitation at 485 nm and emission
at 535 nm was measured with a microplate spectrophotometer
reader Model 680 (BioRad, CA).
Flow cytometry
Flow cytometric analyses were performed as described
elsewhere.16 The following monoclonal antibodies
conjugated to fluorochromes were used: anti-CD29(β1-integrin)-PE,
CD34-PE, CD44-PE, CD49d-PE, CD90(Thy-1)-PE, CD117-PE,
and Tie-2-PE (BD Biosciences, San Diego, CA); CD71-PE
and CD105-PE (Serotec, Oxford, UK); and CD144-PE
(Beckman Coulter, CA, USA).
Statistical analysis
Measured values were expressed as mean ± S.D. Student’s
t-tests were used to compare the population means
between groups when their population variances were
assumed to be equal, whereas Welch’s t-test was
used when variances were unequal. F tests were used
to assess variances of the two groups being compared.
Results
Proliferative capacity of fresh and cryopreserved
ASCs
Doubling times were comparable between fresh and
cryopreserved ASCs (Fig. 1), indicating that cryopreservation
did not affect the proliferative capacity of the
ASCs.
Chondrogenic potential of fresh
and cryopreserved ASCs
The chondrogenic potential of nine lines of ASCs,
consisting of four fresh cell lines and five cryopreserved
lines, was assayed with the micromass culture protocol,
followed by morphometry for quantification (Fig.
2A) and safranin O staining for qualitative analysis
(Fig. 2B). Sizes of micromass, determined by measuring
the areas of the masses using image analysis software,
could be assumed to reflect the capability of chondrogenic
matrix production. Judged based on the micromass
sizes, chondrogenic potentials of fresh and cryopreserved
ASCs were not significantly different. The standard
deviation of micromass sizes of cryopreserved ASCs
cultured in chondrogenic medium was much larger
than that of fresh cells, suggesting variable but
definite effects of long-term cryopreservation on
their chondrogenic potential. Moreover, although
significant differences were detected between chondroinductive
and control culture conditions in each group, the
p value of this difference was lower in the cryopreserved
cell group than in the fresh cell group. Thus, cryopreserved
cells are more strongly influenced by culture medium
than are fresh cells, suggesting that cryopreservation
could exert some effects on ASC susceptibility to
chondrogenic differentiation.
Microphotographs of safranin O-stained sections
of micromass showed higher production of proteoglycan
matrix in cells with chondrogenic induction than
in controls in both fresh and cryopreserved ASCs
(Fig. 2B). As with the observation from the quantification
assay above, micromasses showed comparable degrees
of chondrogenic matrix production in both groups,
but cryopreserved ASCs compared to fresh ASCs appeared
to exhibit a greater sensitivity to the chondroinductive
culture environment.
Osteogenic and adipogenic potential
of ASCs before and after cryopreservation
Fresh and cryopreserved ASCs were assayed for osteogenic
(Fig. 3) and adipogenic (Fig. 4) potential. Osteogenic
potential, determined as calcium deposition in intra-
and extra-cellular spaces, was comparable between
fresh and cryopreserved ASCs; in fact, cryopreserved
cells surpassed fresh cells. Adipogenic potential,
determined based on spectrophotometric absorbance
using AdipoRed staining, showed no apparent difference
between fresh and cryopreserved ASCs. These results
indicate that long-term cryopreservation up to 6
months does not affect the osteogenic and adipogenic
potential of ASCs.
Flow cytometric analysis of cell
surface markers on ASCs
Cell surface marker expression is closely related
to cell lineage and biological properties, including
multipotentiality. Expression profiles of cell surface
markers, as well as their change over passages,
was analyzed with flow cytometry. ASCs before and
after 6 months of cryopreservation showed similar
expression patterns of the cell surface markers
selected for evaluation at all passage numbers examined
(i.e., passages 0, 1, 2, 3, and 7). Also, sequential
changes in the expression patterns were quite similar
between the two groups of ASCs. Only CD34 declined
with increased passage number; the other markers
generally remained constant in both groups (Fig.
5 and Table 1). Therefore, as far as those analyzed
marker subsets are concerned, the expression profile
of cell surface markers of ASCs underwent little
change through cryopreservation.
Discussion
In this study, we demonstrated that human ASCs preserve
their proliferative capacity, mesenchymal multipotency
(remaining chondrogenic, osteogenic, and adipogenic),
and surface marker expression profiles after 6 months
of cryopreservation. The only difference we detected
between fresh and cryopreserved ASCs in this study
was the larger variability in chondrogenic differentiation
potentials of cryopreserved ASCs. Although the results
do not guarantee the validity of preservation longer
than 6 months for ASCs, it is clinically of great
importance that at least a single cycle of freezing,
thawing, and storage at ?196°C up to 6 months does
not affect the biological characteristics of human
ASCs vital for potential cell therapies.
Human fat is readily obtained from liposuction and
frequently used as a filler material for soft tissue
augmentation.19,20 Because multiple lipoinjection
is frequently necessary for maximizing cosmetic
results, some cosmetic surgeons are strongly inclined
to store and repeatedly use the harvested fat. Thus,
cryopreserved human fat tissue has been intensively
investigated,21,22 but there have been few reports
about the effects of cryopreservation on ASCs.23-26
The three reports by Thirumalas et al.23-25 studying
human ASCs as isolated cells mainly focused on the
physical effect of freezing on cell membrane integrity;
another report26 focused on ASC yield from cryopreserved
fat. To our knowledge, prior to the present study
there have been no reports investigating the potential
of cryopreserved human ASCs as a tool for cell therapy.
With advances in tissue engineering and regenerative
medicine, use of adult stem cells may be a solid
therapeutic option in the near future, and our data
can contribute to developing protocols for regenerative
therapies with cryopreserved ASCs. As for other
types of adult stem cells, e.g., hematopoietic stem
cells and umbilical cord blood cells, standard cryopreservation
protocols have been established, and the safety
of long-term storage has been demonstrated.27,28
Based on the findings regarding these antecedent
stem cells, it is likely that we can reasonably
extend the period of cryopreservation of ASCs, though
the safety of doing so should be further examined.
In this study, the influences of different cryoprotective
agents and cryopreservation methods were not examined.
The cryoprotective agent used in this study contains
FBS as a supplement, which contributes to the maintenance
of viability of ASCs during freezing and thawing.
Given the point-of-view that animal-derived biological
ingredients should be completely eliminated from
the process for cells to be used clinically in cell
therapy, the cryoprotective agent may have to be
further optimized for ASC storage.
FIGURE LEGENDS
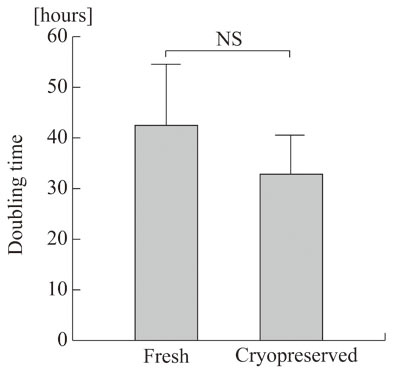
Figure 1.
Doubling time of fresh and cryopreserved human ASCs.
Five lines of human ASCs before the freezing and
thawing process (Fresh) and four lines harvested
after 6 months of cryopreservation (Cryopreserved)
were assayed for cell proliferation rate, and their
doubling times were determined. NS: no significant
difference. Values are mean + S.D.
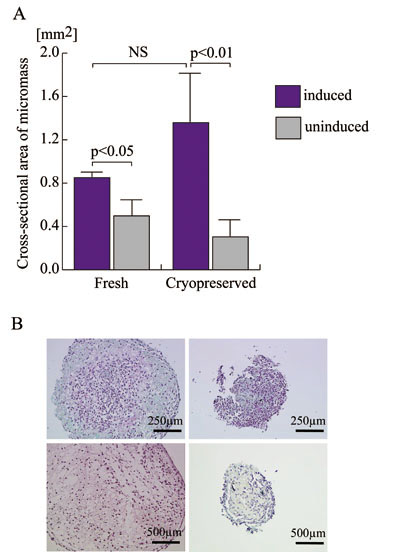
Figure 2.
Chondrogenic potentials of fresh and cryopreserved
human ASCs.
A. Four lines of human ASCs prior to the freezing
and thawing process (Fresh) and five lines harvested
after being cryopreserved for 6 months (Cryopreserved)
were subjected to micromass culture in the defined
chondrogenic differentiation medium (blue columns)
or control medium (grey columns) to assess chondrogenic
potential. Micromass sizes were determined using
image analysis software on microphotographs with
scale as an area [mm2]. NS: no significant difference.
B. Microphotographs of representative sections of
micromass. Paraffin-embedded sections were stained
with safranin-O and counterstained with hematoxylin:
fresh ASCs in chondrogenic medium (above left),
fresh ASCs in control medium (above right), cryopreserved
ASCs in chondrogenic medium (below left), and cryopreserved
ASCs in control medium (below right).
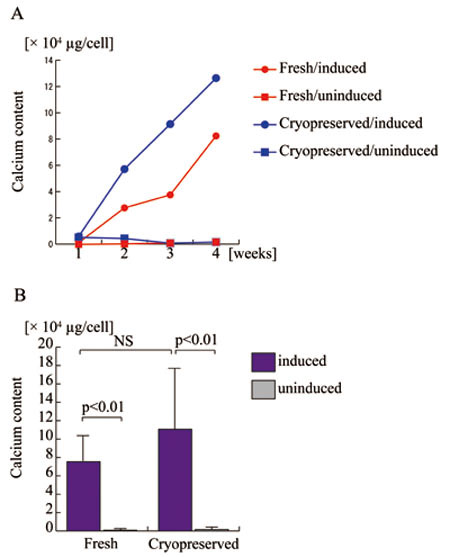
Figure 3.
Osteogenic differentiation potentials of human adipose-derived
stem cells (ASCs) before and after long-term cryopreservation.
A. Fresh (red lines) and cryopreserved (blue lines)
ASCs derived from a single patient were assayed
for calcium deposition after 1, 2, 3, and 4 weeks
of cell culture with osteogenic differentiation
medium (closed circles) or control medium (closed
rectangles). Fresh ASCs with (Fresh/induced) or
without (Fresh/uninduced) osteogenic induction are
indicated with red circles and red rectangles, respectively;
cryopreserved ASCs with (Cryopreserved/induced)
or without (Cryopreserved/induced) osteogenic induction
are indicated with blue circles and blue rectangles,
respectively.
B. Five lines of human ASCs prior to the freezing
and thawing process (Fresh) and four lines after
cryopreservation for 6 months (Cryopreserved) were
assayed for calcium deposition to assess osteogenic
potential with (blue columns) or without (grey columns)
osteogenic induction. NS: no significant difference.
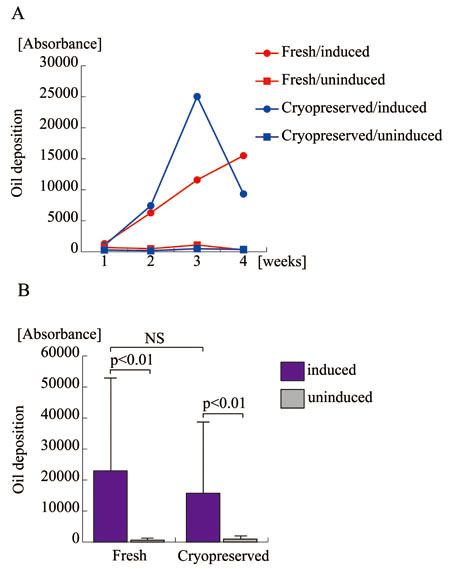
Figure 4.
Adipogenic differentiation potentials of human ASCs
before and after long-term cryopreservation.
A. Fresh and cryopreserved ASCs derived from the
same patient were assayed for oil deposition as
a quantification of adipogenic differentiation at
the indicated time point. Fresh ASCs with (Fresh/induced)
or without (Fresh/uninduced) osteogenic induction
are indicated with red circles and red rectangles,
respectively; cryopreserved ASCs with (Cryopreserved/induced)
or without (Cryopreserved/induced) osteogenic induction
are indicated with blue circles and blue rectangles,
respectively.
B. Five lines of human ASCs prior to the freezing
and thawing process (Fresh) and four lines after
cryopreservation for 6 months (Cryopreserved) were
assayed for lipid deposition to assess adipogenic
potential with (blue columns) or without (grey columns)
adipogenic induction. NS: no significant difference.
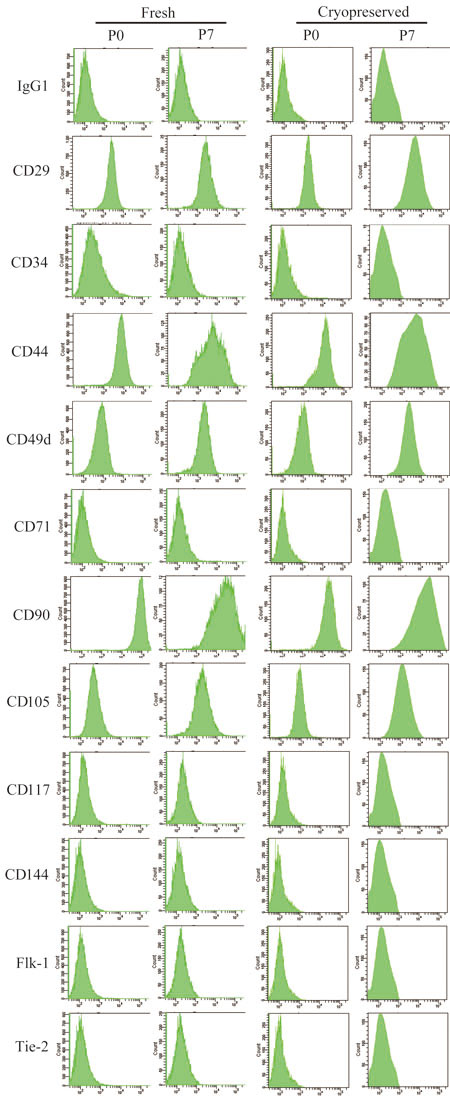
Fig. 5.
Representative data of cell surface marker expression
in fresh and cryopreserved ASCs (passages 0 and
7) obtained from a single patient.
ASCs before (Fresh) and after 6 months of cryopreservation
(Cryopreserved) were analyzed at passages 0 and
7 with flow cytometry for expression of a selected
set of cell surface markers. The representative
data from fresh and cryopreserved ASCs (passages
0 and 7) obtained from a single patient are shown.
IgG1 indicates the negative control using a non-specific
mouse immunoglobulin G1 species as an antibody to
determine background fluorescence.
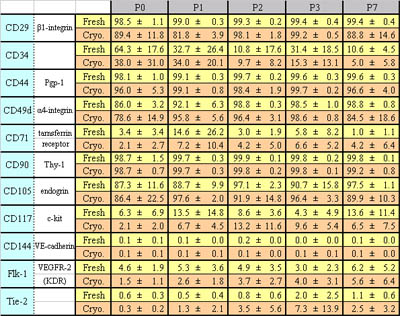
Table 1
Expression of cell surface markers in fresh and
cryopreserved human ASCs.
Percentages of positive cells for each surface marker
are shown. Data were collected from four fresh lines
and five cryopreserved lines; one line from the
fresh and one from the cryopreserved were derived
from the same patient; the others were derived from
different patients. Values are mean ± S.D.
REFERENCES
1. Zuk, P. A., Zhu, M., Ashjian, P., et al. Human
adipose tissue is a source of multipotent stem cells.
Mol. Biol. Cell 13: 4279, 2002.
2. Zuk, P. A., Zhu, M., Mizuno, H., et al. Multilineage
cells from human adipose tissue: implications for
cell-based therapies. Tissue Eng. 7: 211, 2001.
3. Matsumoto, D., Sato, K., Gonda, K., et al. Cell-assisted
lipotransfer (CAL): supportive use of human adipose-derived
cells for soft tissue augmentation with lipoinjection.
Tissue Eng., in press, 2006.
4. Halvorsen, Y. C., Wilkison, W. O. and Gimble,
J. M. Adipose-derived stromal cells--their utility
and potential in bone formation. Int. J. Obes. Relat.
Metab. Disord. 24 Suppl 4: S41, 2000.
5. Dragoo, J. L., Samimi, B., Zhu, M., et al. Tissue-engineered
cartilage and bone using stem cells from human infrapatellar
fat pads. J. Bone. Joint. Surg. Br. 85: 740, 2003.
6. Cowan, C. M., Shi, Y. Y., Aalami, O. O., et al.
Adipose-derived adult stromal cells heal critical-size
mouse calvarial defects. Nat. Biotechnol. 22: 560,
2004.
7. Hicok, K. C., Du Laney, T. V., Zhou, Y. S., et
al. Human adipose-derived adult stem cells produce
osteoid in vivo. Tissue Eng. 10: 371, 2004.
8. Peterson, B., Zhang, J., Iglesias, R., et al.
Healing of critically sized femoral defects, using
genetically modified mesenchymal stem cells from
human adipose tissue. Tissue Eng. 11: 120, 2005.
9. Erickson, G. R., Gimble, J. M., Franklin, D.
M., et al. Chondrogenic potential of adipose tissue-derived
stromal cells in vitro and in vivo. Biochem. Biophys.
Res. Commun. 290: 763, 2002.
10. Awad, H. A., Halvorsen, Y. D., Gimble, J. M.,
et al. Effects of transforming growth factor beta1
and dexamethasone on the growth and chondrogenic
differentiation of adipose-derived stromal cells.
Tissue Eng. 9: 1301, 2003.
11. Huang, J. I., Zuk, P. A., Jones, N. F., et al.
Chondrogenic potential of multipotential cells from
human adipose tissue. Plast. Reconstr. Surg. 113:
585, 2004.
12. Planat-Benard, V., Silvestre, J. S., Cousin,
B., et al. Plasticity of human adipose lineage cells
toward endothelial cells: physiological and therapeutic
perspectives. Circulation 109: 656, 2004.
13. Miranville, A., Heeschen, C., Sengenes, C.,
et al. Improvement of postnatal neovascularization
by human adipose tissue-derived stem cells. Circulation
110: 349, 2004.
14. Cao, Y., Sun, Z., Liao, L., et al. Human adipose
tissue-derived stem cells differentiate into endothelial
cells in vitro and improve postnatal neovascularization
in vivo. Biochem. Biophys. Res. Commun. 332: 370,
2005.
15. Hubel, A. Parameters of cell freezing: implications
for the cryopreservation of stem cells. Transfus.
Med. Rev. 11: 224, 1997.
16. Yoshimura, K., Shigeura, T., Matsumoto, D.,
et al. Characterization of freshly isolated and
cultured cells derived from the fatty and fluid
portions of liposuction aspirates. J. Cell. Physiol.
208: 64, 2006.
17. Johnstone, B., Hering, T.M., Caplan, A.I., et
al. In vitro chondrogenesis of bone marrow-derived
mesenchymal progenitor cells. Exp. Cell Res. 238:
265, 1998.
18. Connerty, H. V. and Briggs, A. R. Determination
of serum calcium by means of orthocresolphthalein
complexone. Am. J. Clin. Pathol. 45: 290, 1966.
19. Fulton, J. E., Suarez, M., Silverton, K., et
al. Small volume fat transfer. Dermatol. Surg. 24:
857, 1998.
20. Moscatello, D. K., Dougherty, M., Narins, R.
S., et al. Cryopreservation of human fat for soft
tissue augmentation: viability requires use of cryoprotectant
and controlled freezing and storage. Dermatol. Surg.
31: 1506, 2005.
21. Ullmann, Y., Shoshani, O., Fodor, L., et al.
Long-term fat preservation. J. Drugs Dermatol. 3:
266, 2004.
22. Shoshani, O., Ullmann, Y., Shupak, A., et al.
The role of frozen storage in preserving adipose
tissue obtained by suction-assisted lipectomy for
repeated fat injection procedures. Dermatol. Surg.
27: 645, 2001.
23. Thirumala, S., Gimble, J. M. and Devireddy,
R. V. Transport phenomena during freezing of adipose
tissue derived adult stem cells. Biotechnol. Bioeng.
92: 372, 2005.
24. Thirumala, S., Zvonic, S., Floyd, E., et al.
Effect of various freezing parameters on the immediate
post-thaw membrane integrity of adipose tissue derived
adult stem cells. Biotechnol. Prog. 21: 1511, 2005.
25. Devireddy, R. V., Thirumala, S. and Gimble,
J. M. Cellular response of adipose derived passage-4
adult stem cells to freezing stress. J. Biomech.
Eng. 127: 1081, 2005.
26. Pu, L. L., Cui, X., Fink, B. F., et al. Adipose
aspirates as a source for human processed lipoaspirate
cells after optimal cryopreservation. Plast. Reconstr.
Surg. 117: 1845, 2006.
27. Rowley, S. D. Hematopoietic stem cell cryopreservation:
a review of current techniques. J. Hematother. 1:
233, 1992.
28. Kobylka, P., Ivanyi, P. and Breur-Vriesendorp,
B. S. Preservation of immunological and colony-forming
capacities of long-term (15 years) cryopreserved
cord blood cells. Transplantation 65: 1275, 1998.